Many researchers have heard about Raman spectroscopy, yet it is often less common knowledge where the technique originated and how it works. This is all the more important because even though new applications increase at a rapid pace, the underlying fundamental principles remain the same.
When photons pass through a material, a fraction of them change direction through a process called light scattering. For transparent materials, most of this scattering is elastic through a process called Rayleigh scattering, which changes the direction of the light but not its wavelength.
In 1928 the Indian physicist C. V. Raman and his student K. S. Krishnan observed that a tiny fraction of light passing through a transparent material can also scatter inelastically, meaning that the scattered light has a different wavelength than the incoming light. This type of scattering is now also called Raman scattering. Soon after their initial experiments, the researchers realised that they could use the spectrum of inelastically scattered photons to identify different chemical compounds.
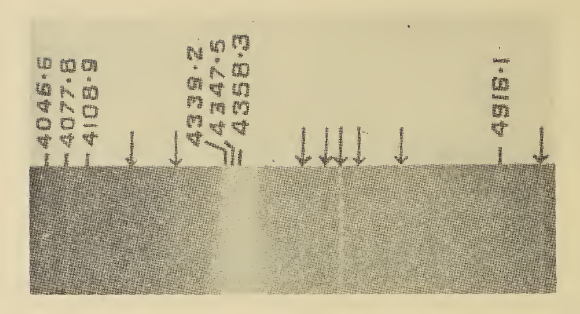
Raman spectrum of Benzene, Raman, C., Krishnan, K. The Negative Absorption of Radiation. Nature 122, 12–13 (1928). https://doi.org/10.1038/122012b0
The shift in wavelength in the inelastic scattering process is related to a change in vibrational states of chemical bonds inside the material. The accompanying shifts in wavelength depends on the the chemical and physical properties of the material and are therefore be used as unique fingerprints. The figure above shows their recorded spectrum for benzene.
Since its inception, Raman spectroscopy has become an increasingly important analysis tool in research laboratories and industrial processes. In modern Raman spectrometers, a sample is exposed to a monochromatic light source that is typically provided by a laser.
Advantages and disadvantages compared to traditional IR spectroscopy
Traditional IR spectroscopy relies on the absorption of infrared light photons. This absorption happens when the wavelength of the incoming light corresponds to the difference of two vibrational energy states for one or more chemical bonds. Upon absorption, a photon is annihilated and its energy is transferred to accommodate for the energy shift. Different compounds can be identified by their spectrum showing the amount of light that is absorbed at a range of different wavelengths.
Transitions that show up weakly in traditional IR, may still be strongly present in Raman scattering. This is because the tendency of molecules that exhibit Raman scattering is proportional to their tendency to change their polarisability as a function of vibrational coordinates. Even for materials that are susceptible to Raman scattering, only a tiny fraction of the incoming light scatters inelastically. Therefore, the incident light beam needs to be sufficiently strong and the detectors used need to be sensitive in order to collect a enough number of inelastically scattered photons over a range of wavelengths that is required to record a spectrum. Also, appropriate filters must be used to filter out the elastically (Rayleigh) scattered light. For this reason, Raman spectroscopy equipment is typically relatively costly. Also, the heat generated by the laser can harm sensitive samples.
One great advantage of Raman spectroscopy is the possibility to analyse aqueous samples. In traditional IR spectroscopy this is more difficult because water strongly absorbs infrared radiation over a broad range of wavelengths. In Raman spectroscopy this not a problem because water molecules are not so susceptible to Raman scattering.
A multifaceted technology
Raman spectrometers are routinely used as laboratory equipment to analyse substances and measure their chemicals fingerprint. Because it is typically not required to record a background signal, the techniques can be even used to quickly analyse substances kept inside glass bottles and containers to determine their nature, purity of quality of substances.
Raman spectroscopy can also be used to measure the crystallographic orientation of crystalline materials or obtain information on the composition of advanced materials through measuring characteristic phonon modes. For example, Raman spectroscopy can be used to measure stresses and strains inside materials.
Recent advances
An interesting development is that Raman spectrometers are becoming more integrated. One example is their use in Raman microspectroscopy, combining both features from microscopy and spectroscopy to create images. When the excitation beam is aimed at a field of view, the presence of specific compounds in different regions can be detected with a CCD or PMT through their Raman peaks at characteristic wavelengths. In this way it is possible to make spectral maps with relative concentrations of molecules in different areas. Other interesting developments can be found in new ways to enhance signals from Raman scattering by using resonance techniques, electric fields, and tips and sensors made out of novel materials and supraparticles.
Some modern instruments also integrate Raman spectroscopy within confocal microscopy such as here and here, which allows for making Raman 3D reconstructions of samples and accurately map the spatial distribution of physical and chemical properties in microscopic detail.
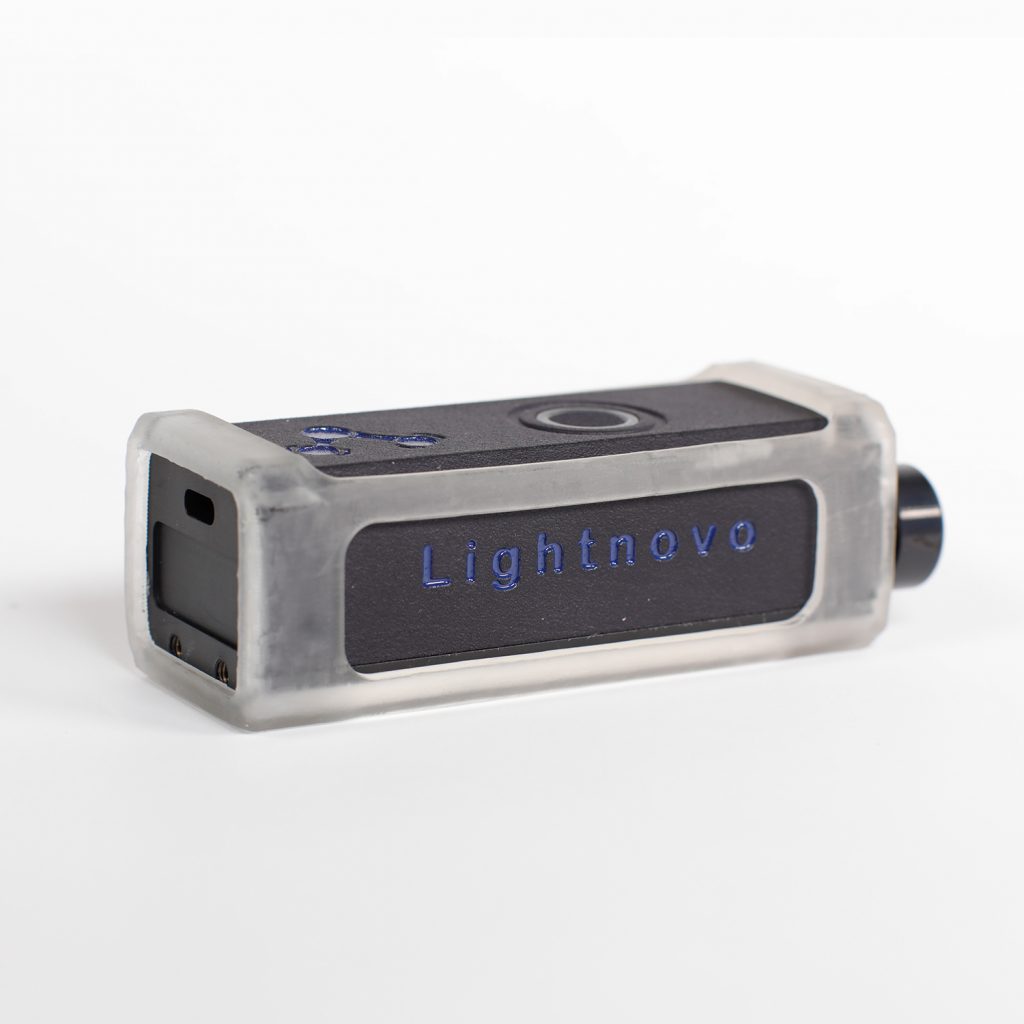
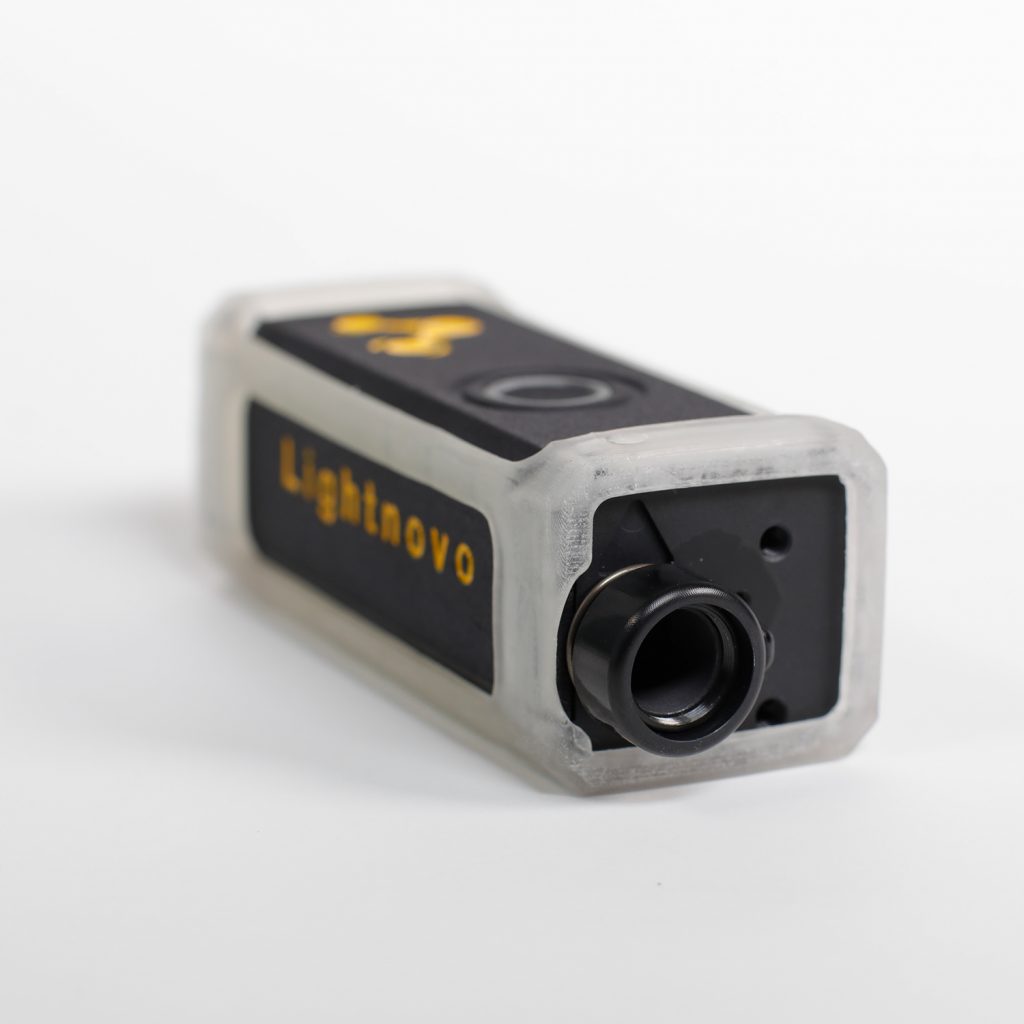
Because Raman spectroscopy has traditionally been a relatively expensive analysis technique, a new and interesting trend is to make Raman spectrometers more portable and affordable laboratory equipment. For example, Lightnovo has successfully developed miniaturised Raman spectrometers. Common applications are Surface Enhanced Raman Spectroscopy (SERS), chemical analysis, and forensic analysis. The MiniRaman spectrometers can also be integrated into small (confocal) microscopes that can be used for in-vivo diagnostics, drug detection and 3D Raman imaging.
Outlook
Raman spectroscopy is a useful and versatile tool, and it is interesting to see how it has developed over the last century. When the first spectra were recorded, the process took hours to days because of weak light sources, insensitive detectors and weak Raman scattering cross-sections of the materials inside the samples. Nowadays, spectra can be recorded in a matter of seconds and the technique is integrated with many other powerful analysis tools in microscopy and other advanced instrumentation. The growing number of applications and integrations of Raman spectroscopy and the fact that the technique also becomes increasingly affordable and portable holds promise for increased adaption in laboratories and industrial settings around the world. Exciting indeed!